CERECAM’s Research Strategy (2025-2030) outlines the centre's vision for advancing computational and applied mechanics in Africa. This strategy highlights the Centre’s commitment to cutting-edge, interdisciplinary research that bridges fundamental theories with real-world applications. By leveraging computational methods, advanced simulations, and data-driven approaches, CERECAM addresses challenges in biomedical science, structural engineering, materials development, and environmental sustainability. Our research fosters innovation while strengthening Africa’s capacity in computational and applied mechanics, ensuring local expertise contributes to global scientific advancements.
Read the full strategy here.
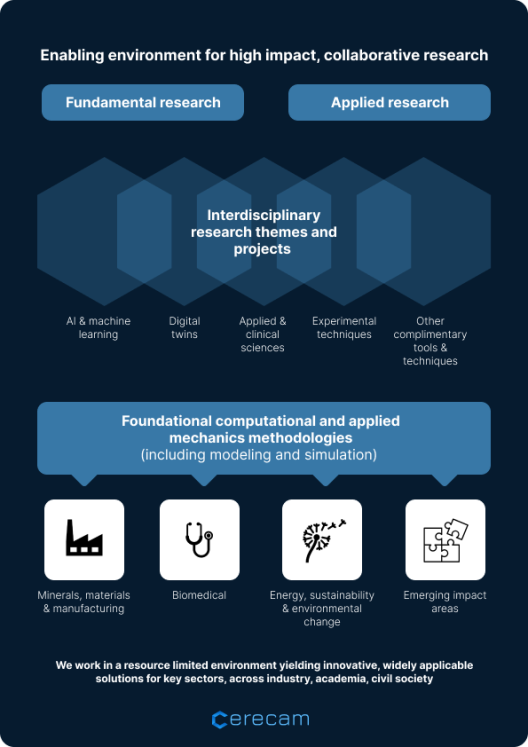
Research activities in CERECAM are organised into a number of research programmes ranging from fundamental research to projects with a direct link to industrial and other applications. View our key publications here.
This theme encompasses a broad range of activities, covering the span from theoretical to applied research. This includes topics in strain-gradient plasticity, aluminium processing and Antarctic sea ice.
There has been sustained activity at CERECAM in the area of strain-gradient plasticity. The first works were concerned with the development of a rate-independent model with an associative flow relation and yield criterion in microstress space. These works also developed variational formulations of the problem and presented conditions for well-posedness of solutions. An important aspect of models of strain-gradient plasticity is in the form of the defect energy included in the free energy. Various forms of this energy have been proposed in the literature. Using the defect energy proposed by Gurtin and Anand, some mathematical analysis has been explored. Models of an alternative approach that relate size-dependent responses to gradients in stress, have been motivated, proposed and explored in recent years.
Improved understanding of material responses can inform industrial processes. During the hot-rolling of certain aluminium alloys, rolling forces are significantly higher than process modelling based on existing material model predictions. This work aims to develop material models that accurately predict rolling forces by taking the progressive breakdown of inter-metallic particles present in the metal into account. Models are built on data obtained from high-temperature experiments performed at representative strain rates. The work is done in collaboration with an industrial partner Hulamin, a semi-fabricator of aluminium products.
An improved understanding of material responses is also beneficial for environmental conservation, Antarctic sea-ice dynamics are poorly understood, because they are significantly different from those observed in the Arctic, where most research efforts have been focused. Therefore, an interdisciplinary research collaboration has been established between the Department of Civil Engineering and the UCT Departments of Oceanography, Chemical Engineering and Electrical Engineering to combine observations from satellite and deployed buoys, in-situ ice sampling and testing during dedicated expeditions, lab experiments and numerical modelling in an effort to unravel the complex processes in the Antarctic marginal ice zone governing, the mechanical interaction of sea ice with wind, ocean currents and waves; the wave energy dissipation and scattering as linked to ice type, ice thickness and floe size; and the thermodynamics of ice growth and brine drainage.
Focus Areas: Strain-gradient plasticity, emphasizing length scales and non-local gradient terms to understand size dependence and dislocation behaviors; Aluminium processing; Seasonal and long-term changes in Antarctic sea ice and shelf ice
Impact: Development of models capturing non-proportional loading behaviors and interactions. This theme contributes significantly to the understanding of material behavior under diverse loading conditions, including industrial and environmental applications.
Research in this area has been directed towards the development, analysis and implementation of new finite element and related formulations that are simple but stable and efficient, particularly in situations involving small parameters such as those that arise in situations of near incompressibility and near-inextensibility. The focus has been to undertake detailed analyses and to generate novel approaches, largely within the context of the discontinuous Galerkin (DG) and virtual element methods (VEM). Together with collaborative partners, more recent work has also focused on the development of representative volume elements (RVEs).
Focus Area: Development, analysis, and implementation of new finite elements and related computational methods.
Impact: Enhancing the stability and efficiency of computational models, particularly for materials exhibiting near incompressibility and inextensibility, thereby advancing the field of computational mechanics.
This theme focusses on the application of mechanics principles to the study of biomedical and biological systems, and spans the spectrum of solid and fluid mechanics.
Biomedical projects are often carried out in conjunction with clinical partners and include a range of cardiovascular applications. These include the application of cardiac mechanics to rheumatic heart disease, where the myocardial material response is obtained from the micro-structural level where it originates. Two different avenues are pursued: (i) generalised continuum methods and (ii) FE-square-based modelling. Our work on congenital heart disease focusses on altered haemodynamics in lesions and incorporates fluid flow, growth modelling and machine learning approaches, with a view to contributing to interventional planning. Our thrombosis work also originated from an interventional planning focus in cerebral aneurysms, but has expanded to applications in deep vein thrombosis, COVID and aortic valves. On the treatment end, our work has focused on the modelling of hydrogel injectate therapies for heart attacks with a view to improving our understanding of hydrogel dispersion after injection into the heart tissue. This incorporates modelling of solid and fluid domains.
An understanding of biological material behaviour is important for biomimetic design. For example, many natural protective structures, such as alligator armour, turtle shells, and the skulls of many animals including humans, contain networks of sutures; those are soft tissues that bond adjacent stiff plates typically made of bone. Such protective structures ought to withstand large loads associated with predator attacks. Computational models of turtle shells enable focus on the influence of the network of sutures. The ability of sutures to reduce the maximum strain energy is explored. Another example is keratin fibres, with particular focus on human scalp hair. Straight and curly human hair fibres have the same building blocks but have different mechanical response patterns. An improved understanding of the factors that give rise to these differences could be instructive for material design and keratin-based fibre applications.
Focus areas: Cardiac mechanics; Congenital heart disease; Human hair mechanics; Modelling of injectates for heart attack therapies; Sutures; Thrombosis
Impact: This research provides insights into biomedical and biological problems, potentially informing biomimetic design and improving medical interventions for diseases.
This work is concerned with mathematical modelling and computational solution of Newtonian and non-Newtonian fluid flow. These include isothermal and non-isothermal processes in fluid and polymer systems with applications in engineering and biological systems. Objectives include the development of efficient computational methods of solution as well as comparative studies of the flow response of different fluids in a variety of contexts.
Focus area: Modelling and analysis of complex fluid flows with applications in various industrial and biological systems.
Impact: The research has implications for a wide range of industrial applications, contributing to the design and optimisation of systems like turbine blades and addressing challenges in fluid dynamics.
Particulate flow modelling combines the inherent frictional nature of particles with their distinctly fluid-like structure. Given the high concentration of solids in industrial flows, the granular flow approximation is unique in its ability to capture the solid-solid interactions within a Navier-Stokes-like framework, typical of fluid flow. The physically valid dissipative mechanisms associated with granular material allows for more realistic quantification of abrasion and attrition in comminution processes while accurately capturing important flow features like free surface shape and velocity field profiles.
Industrial systems such as tumbling mills are typified by rotational and axial flows of rock, steel balls and slurry. The tortuous porous network, created by the non-uniform packing of rock and steel balls, form the channels through which the viscous slurry flows. A multi-pronged approach employing non-invasive nuclear techniques (PEPT, X-ray) and computational modelling (DEM, CFD (principally, Finite Elements and/or Finite Volume Methods), SPH) has formed the key ingredients for mechanistic modelling of such industrial systems.
Focus area: Characterisation of granular flow in industrial and biological systems, employing computational modelling and advanced tracking techniques.
Impact: This work is crucial for understanding granular flow dynamics in different contexts, from industrial processes like milling and smelting to biological systems like the human airway.